The JASM Framework
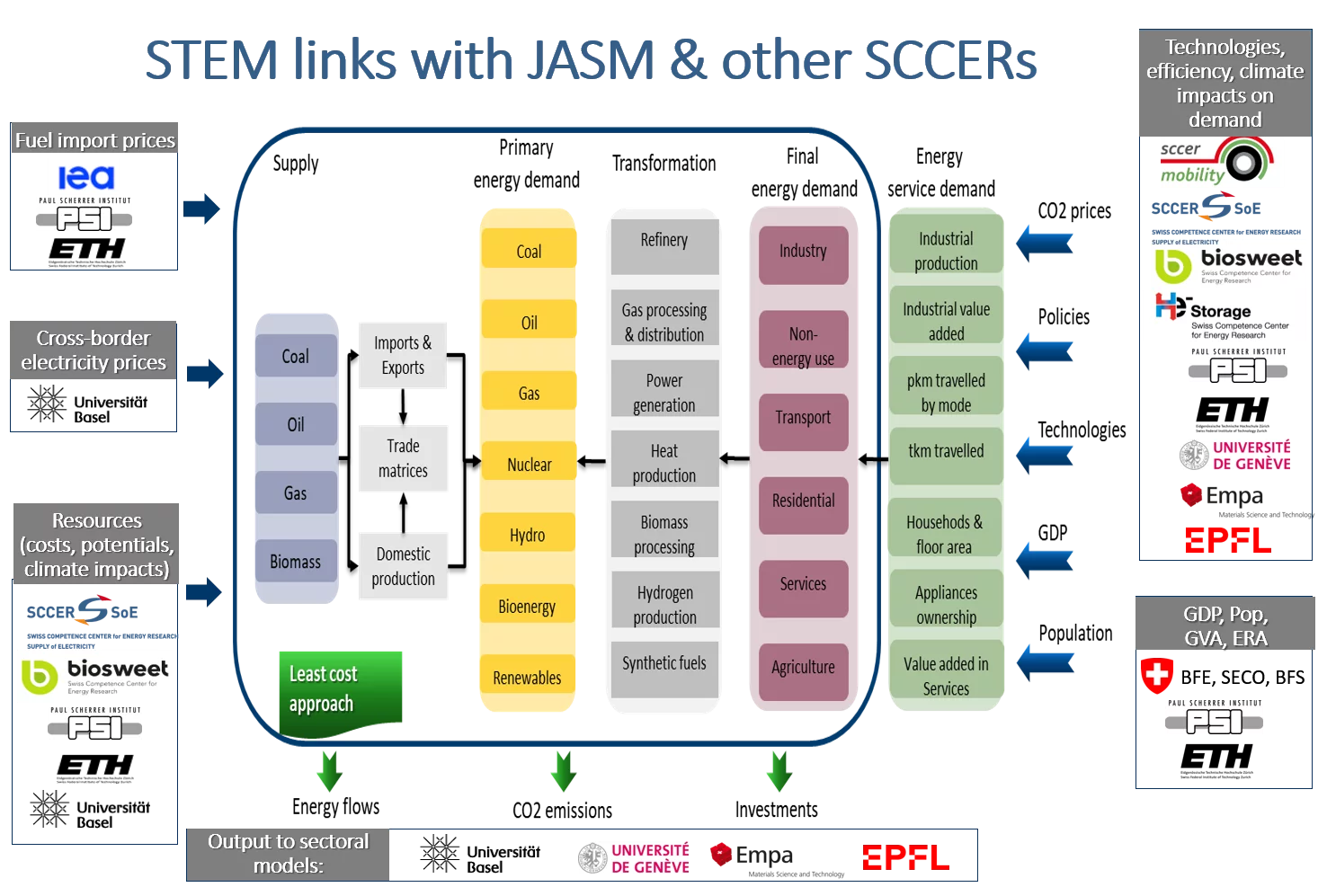
Supply of Electricity (SoE), Efficient Technologies and Systems for Mobility (Mobility), Heat and Electricity Storage (HaE), Biomass for Swiss Energy Future (Biosweet), Society and Transition (CREST), Efficiency of Industrial Processes (EIP), and Future Swiss Electrical Infrastructure (FURIES).
The JASM framework includes three energy-system models: the Swiss TIMES Energy Systems Model (STEM) from PSI, and two versions of the Swiss Energy Scope (SES) from EPFL and ETHZ. The energy systems models are informed by several sectoral models that represent and analyse in detail key sectors of the energy system, such as Swissmod from University of Basel that models the electricity sector and climate impacts on water inflows to hydropower plants, EURO-CORDEX simulations to study the impact of climate change on heating and cooling demands in Switzerland from Hochschule Luzern, stock model that provides hourly load curves for electricity use from University of Geneva, the CESAR model from EMPA to provide load curves for heating and efficiency measures in buildings together with the SWISSRES model from University of Geneva, modelling of energy efficiency in industry from University of Geneva and EPFL, and distribution grid modelling from EPFL to assess PV hosting capacity at medium and low voltage grid levels.
Within the JASM framework STEM of PSI LEA has been greatly improved and further extended regarding the representation of the Swiss energy system. There was an extensive and successful collaboration between the Energy Economics Group of LEA and the teams involved in JASM. STEM focused on assessing challenges and opportunities associated with the clean energy transition in all areas of the energy system and provided insights regarding the sectoral transformation pathways and associated costs.
Long-term scenarios and variants assessed with STEM
The Baseline (short BAU) scenario, assumes a continuation of existing trends in energy consumption and supply, and in the performance of technologies. It does not enforce renewables deployment or climate change mitigation targets. It implements a gradual phase-out of existing nuclear power plants , by assuming a lifetime of 60 years. BAU is primarily used to benchmark the developments and to showcase upcoming challenges in long-term scenarios related to the energy system transition.
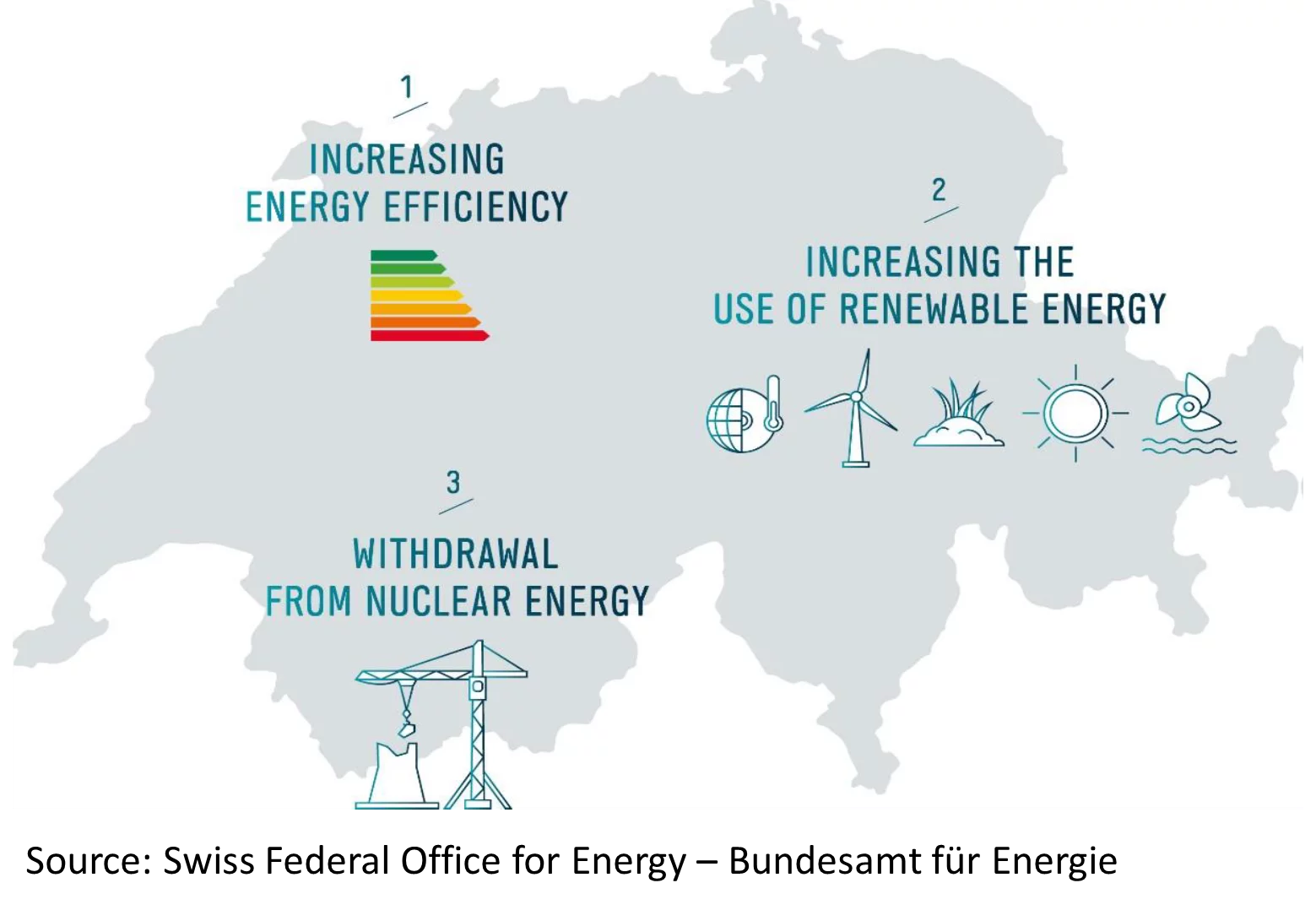
The Energy Policy (short EPOL) scenario takes into account the measures and targets of the Swiss Energy Strategy, which is in force since 2018. It implements both the targets on renewable energy deployment and energy efficiency, as defined in the Swiss Energy Strategy. The energy efficiency includes both targets related to the reduction of overall energy consumption and electricity consumption. However, the EPOL scenario does not impose specific targets in emissions reduction. It can be considered as an explorative scenario regarding the decarbonisation of the Swiss energy system. Although EPOL does not include explicit overall decarbonisation targets, it includes the vehicle CO2 emissions standards in-line with the Swiss Energy Strategy and the standards of the European Commission. In addition, it assumes the interconnection between the Swiss and EU emissions. In addition, it assumes the interconnection between the Swiss and EU emissions trading schemes (ETS), and it implements the post-2020 2.2% linear emission reduction factors announced by the European Commission2. The EPOL scenario has been specifically built to evaluate the abatement level of greenhouse gases (GHG) emissions, which can be achieved with the implementation of efficiency measures towards a well-below 2000Watt society by 2060.
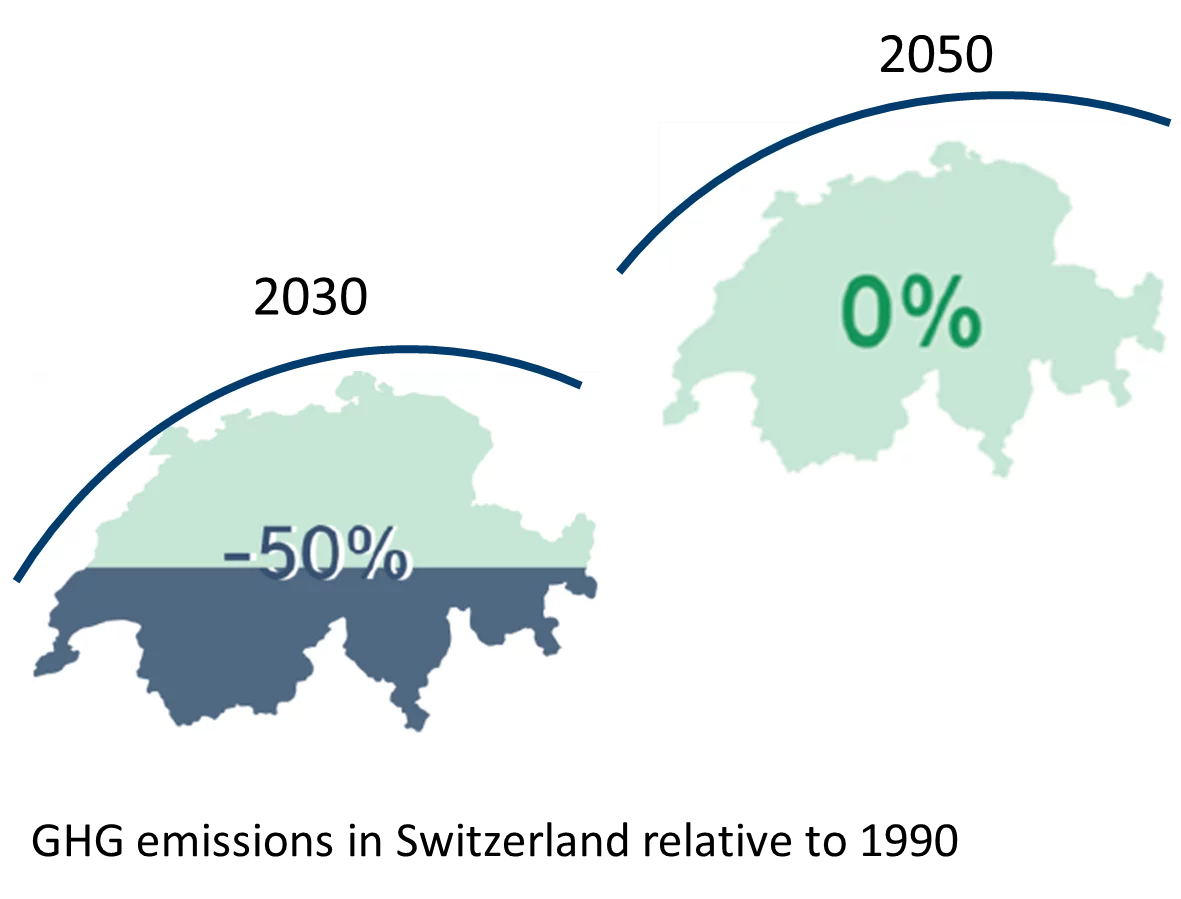
The Net-Zero (short CLI) scenario aims at achieving the target of net-zero emissions in 2050, in a context of phasing-out existing nuclear power plants. The Swiss Federal Council announced this ambition in summer of 2019, and it has been communicated as an indicative target to the UNFCCC in December 2020. The net-zero target in 2050, as the Swiss Federal Council announced, includes energy related emissions, emissions from industrial processes and also non-energy related emissions from agriculture, wastes, land use, land use change and forestry. However, the analysis with STEM focuses only on achieving net-zero emissions in the energy system and industrial processes (i.e. it does not include emissions from agriculture and waste, other than fuel combustion). Besides this, the analysis does not consider compensation of domestic emissions abroad, although imported zero-carbon fuels and energy carriers can be regarded as such. The non-energy emissions could be at least 5 Mt CO2-eq in 2050 of non-CO2 greenhouse gases according to the Swiss Federal Office for Environment. If in the current analysis the energy system were offset these emissions too, the emissions target in CLI should have been set to at least -5 Mt CO2-eq in 2050. The CLI scenario also implements sectoral policy measures and emissions targets described in the revision of the CO2 law, such as emissions standards for buildings and vehicles and promotion of renewable fuels in transport, as well as it also assumes the strengthening of the Swiss and EU emission trading schemes. Beyond 2030, the vehicle emissions standards and ETS targets are based on scenario projections from the European Commission, while for buildings the MINERGIE standards are used.
Fragmented policy (short ANTI). ANTI assumes international context with low cooperation and fragmented policies in mitigating climate change. There are reduced R&D expenditures in low-carbon technologies and weaker integration between Swiss and international energy markets compared to CLI. In this setup of national and international contexts, there is limited tolerance from Swiss society regarding landscape changes for implementing renewable and other low-carbon projects and limited exploitation of the domestic renewable potential, also due to high upfront costs.
Energy security (short SECUR). SECUR puts emphasis on achieving net-zero emissions in 2050 by reducing import dependency to the minimum. SECUR assumes the same international context and weak integration of energy markets as in ANTI, but the Swiss society is keener in using domestic renewable resources and means to reduce emissions. Reinforcement and expansion of domestic infrastructures is socially acceptable and desirable to increase reliability of the energy system, reduce grid congestion and provide flexibility in integrating renewable sources.
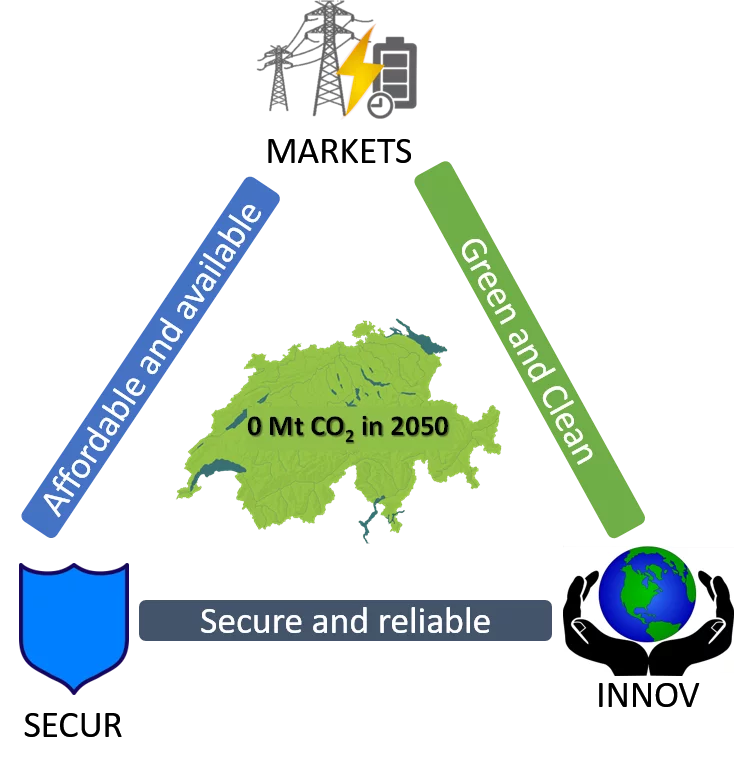
Market integration (short MARKETS). MARKETS emphasises the role of higher integration of Swiss and international energy markets, beyond the levels assumed in CLI. Starting from the social acceptance for using domestic renewable energy sources as in SECUR, priority in MARKETS is the decarbonisation through affordable energy. To achieve it, local markets and "prosumage" schemes are efficiently coordinated with national energy markets. Technologies enabling sector coupling and flexibility enjoy economies of scale and lower costs than in CLI. Reinforcement of domestic and cross-border infrastructures occurs to support market integration, reduce congestion, and increase reliability of the energy system.
Technology innovation (short INNOV). In INNOV governments acknowledge climate change as one of the key future challenges for society. In a global effort to achieve the targets of Paris Agreements, they implement ambitious research, innovation and demonstration programs related to low-carbon technologies, which are also supported with private co-financing. Building on the high market integration and access to domestic renewable resources of MARKETS, INNOV assumes lower costs of clean and low-carbon technologies than CLI. Circular economy reduces the need for materials and results in lower costs for energy conservation measures. Grid reinforcement to mitigate congestion, improve energy markets integration, increase reliability and flexibility of the energy system occurs as well.
EPOL-E: in this variant of EPOL the per capita electricity reduction target is not included
CLI80, CLI100: these two variants of CLI are inspired by the collaboration with the SCCER Mobility. In both CLI80 and CLI100, the emissions standards in vehicles, the emissions standards in buildings, and the ETS linear emissions reduction factor do not become more stringent beyond 2030, but they remain at the levels of 2030 throughout the period of 2030-2050. In CLI80, the emissions reduction target is set to 80% compared to 1990 levels, while in CLI100, the emissions trajectory is the same as in the CLI scenario. Since the CLI100 variant does not implement the whole spectrum of policies described in the revised CO2 law, it can be regarded as quite close to the Least Cost solution, in which the model is free to take decisions, constrained only by the overarching emissions reduction targets in 2030 and 2050.
Key findings
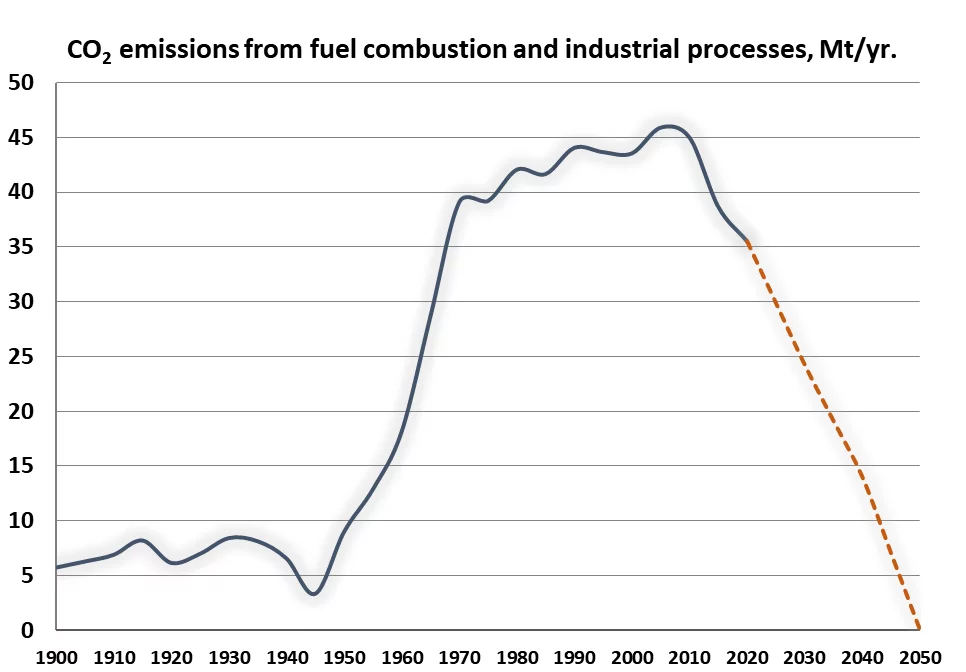
The installed capacity of solar PV power needs to double almost every decade from now to 2050. The private car fleet would need to be mostly based on electric drive trains by 2050, which means one in every three new car registrations must be electric by 2030. The deployment of heat pumps needs to accelerate in services and residential sectors so that, by 2050, heat pumps would cover close to three quarters of the space and water heating demand in buildings. Also, it would be necessary to reap efficiency gains by rolling out energy saving measures.
In achieving the net-zero ambition, the total electricity consumption of the energy end-use sectors (industry, residential, services and transport) increases by 11 TWh in 2050 from 2019 levels. This growth is mainly driven by using electricity to power cars, buses, and trucks. By also accounting the electricity used for producing hydrogen and synthetic e-fuels consumed mainly in transport, the total domestic electricity consumption increases by around 20 TWh between 2019 and 2050. In the stationary sectors, electricity becomes increasingly important as heat pumps are deployed more widely. After 2030, efficiency gains offset increased consumption, and the stationary sectors show a plateauing electricity consumption. Electrification and efficiency improvements enable a reduction of the final energy consumption per capita by 55% in 2050 compared to 2000 levels, slightly higher than the long-term target of the Swiss Energy Strategy
Hydrogen penetrates industry and mobility sectors for applications where the direct use of electricity is challenging or associated with very high costs. Long-distance public and freight road transport and heavy industry, are hardest to decarbonise and provide prospects for new hydrogen technologies. About 11 TWh of hydrogen (half of it produced via electrolysis) are used directly, or indirectly for producing synthetic fuels, in 2050. Imported biofuels and synthetic fuels of about 10 TWh in total are also needed by 2050, mainly in the long-distance passenger and freight road transport.
Achieving the net-zero goal in a cost-efficient way would require capturing about 8.6 Mt CO2 from the energy system in 2050. In this regard, capture, utilisation and storage of CO2 is a crucial mitigation option to reach deep decarbonisation. About half of the captured emissions are from negative emissions technologies such as bioenergy with carbon capture and direct air capture. Bioenergy conversion with carbon capture can become vital not only for removing CO2 from the atmosphere but also for producing hydrogen which is also directly used to replace fossil fuels. Failing to harvest the remaining exploitable sustainable potential of bioenergy would entail high climate change mitigation costs. Also, if storing captured CO2 in Switzerland is a challenge, access to international CO2 transport and storage infrastructure would be essential to avoid a drastic increase in mitigation costs.
In 2050, the remaining emissions are in industry and relate to processes that need gaseous and solid (such as waste) fuels. The penetration of alternative vehicles, heat pumps and efficiency measures via renovation to fully decarbonize transport and buildings implies that more than two-thirds of the emissions reduction required to achieve the net-zero emissions goal stem from technologies that are already commercially available or under demonstration. To scale-up their deployment and to transform the entire energy system will require progress across a wide range of technologies and action across all sectors, not just electricity. Long-term policy targets need to be backed up by detailed, clean energy technology strategies that involve measures tailored to local infrastructure and technology integration needs, and that achieve high levels of social acceptance of the new technologies.
The cost of the transition of the Swiss energy system to net-zero emissions in 2050 depends on the resource availability, social acceptance, technology progress, and the level of integration of local, national and international markets. It is also affected by the mitigation level already achieved in the reference scenario, against which the policy cost is calculated. The developments in the aforementioned factors reveal a large cost range, which indicates the uncertainty range of the abatement effort.
In the net zero main scenario assumed in the study, the average discounted additional costs of the climate protection scenario compared to the reference scenario with moderate climate protection (40% CO2 reduction in 2050 compared to 1990) in Switzerland would amount to around 330 CHF per person per year (basis 2010) for the period up to 2050. Looking at all of the scenarios examined, one can see a range of average costs between 200 and 860 CHF2010 per person per year, which ultimately reflects different developments in energy technologies, resource availability, and market integration, in the acceptance of technologies, and in preferences regarding supply security.
It should be noted that the per capita costs are averaged over the investigated time horizon until 2050, which is characterised by a fundamental transformation of the energy system and new technology and fuel mixes. The associated annual policy costs increase exponentially over the projection period, meaning that by 2050 we have built a more expensive energy system, which will require endured investments and expenditures for low carbon energy supply and demand also beyond 2050.